Abstract
Fasteners are widely used in automobile, electronic and other fields, and the demand for high performance fastener materials is increasingly urgent. 10B21 mild steel has the advantages of good plasticity, obvious heat treatment effect and so on. It is used to produce fasteners below grade 9.8. In this paper, the domestic 10B21 boron steel as the research object, through quenching, tempering and other heat treatment, change its structure from ferrite to tempered martensite, in order to improve the strength and hardness.
1. Introduction
With the development of automobile industry, the development and application of high strength auto fasteners have attracted much attention. The raw materials used to produce fasteners are more demanding. Boron steel has plenty of advantages, such as the stable composition, low harmful elements, high purity of steel, small decarburization layer, less surface defects, low cold heading cracking, low original hardness of wire rod, high strength after heat treatment and good hardenability, etc. It has good application prospect in the automobile industry [1-3]. Among them, 10B21 is a typical representative, which has good plasticity, cold heading performance and better hardenability. The spheroidizing annealing treatment of medium carbon steel bolts can be omitted. It is one of the main materials for high strength bolts. Because of its low cost and good machining performance, 10B21 boron steel has become a research hotspot in the field of automotive high strength fasteners. B element plays an important role in alloying 10B21 boron steel, which can improve the hardenability of steel [4-7]. Therefore, the quenchable size of steel can be increased, or the uniformity of microstructure and properties in the quenched steel section can be improved.
Studies have shown that [8-10], the reason why alloying elements can improve the comprehensive mechanical properties of steel is that they can strengthen ferrite by solid solution, increase the recrystallization temperature of ferrite, especially slow down or prevent carbide precipitation and aggregation during tempering, so that cold heading steel has higher tempering resistance. The alloying elements can improve the hardenability of steel mainly because they prevent the transformation of austenite into proeutectoid ferrite, pearlite or upper bainite during quenching. Dong et al. [7, 11-12] explored Trace B is isolated at the boundary, occupies the appropriate nucleation position in advance, reduces the austenite grain boundary energy, inhibits the ferrite nucleation and delays the ferrite formation. At the same time, the boron atom on the grain boundary also blocks the diffusion of the grain boundary atom, and slows down the ferrite nucleation on the grain boundary, thus improving the thermoplasticity of the material. In addition, boron in carbon-boron steel moves fast. During the cooling process, boron can quickly fill in the lattice defects such as dislocation and atomic voids, thus reducing the deformation of grain boundaries and making the grain boundaries stable. Boron occupies the voids of ferrite, and preferential segregation occurs at the grain boundary, which strengthens the grain boundary to some extent. The addition of trace elements such as B is beneficial to prevent the initiation and propagation of the delayed fracture crack grain boundary. With the increase of tensile strength, the ratio of delayed breaking strength of bolt tends to decrease [13-14]. In order to obtain the best microstructure and meet the performance and quality requirements of fasteners, heat treatment is particularly important.
2. Experimental design and implementation
The specific implementation of the experiment is described later. The experimental samples are M8 × 30 bolts which are processed by cold heading forming of 10B21 wire rod. and its chemical composition (mass fraction, %) is: C 0.18-0.23; Si ≤0.10; Mn 0.70-1.00; 0.030; 0.035; 0.008, trace amounts of Mo, V and Cr, the balance being Fe. The experimental material is heat treated. First, the samples were treated with oil removal, phosphorus removal, hot water washing, drying, and quenching heating (870 °C, held about 85 min) and quenching cooling in oil (60 °C). Then, the samples were tempered (440~450 °C, held about 120 min) and water cooling (50 °C). After that, the experimental material is cut into a sample block and number it. Next, use different types of silicon carbide sandpaper to polish the sample step by step to the mirror surface. Finally, perform a continuous cold air drying process.
The 10B21 boron steel samples were mechanically polished, etched in nitrate alcohol solution (5vol.% HNO3), cleaned (deionized water) and dried by cold air to observe the microstructure. The microstructure of the samples was observed by LECAM 5000 metallographic microscope.
The hardness test, tensile test, torque, clamping force were experimented among before and after 10B21 heat treatment samples to explore the mechanical properties changes. Many samples were tested tensile according to GB/tb228-2010 standard, it was carried out by WA-600 universal testing machine. The average value of tensile test was obtained. The sample was polished to a smooth mirror surface by mechanical polishing to test hardness through HV-1000A microhardness tester with a load of 0.3 kgf and a load holding time of 10 s. The hardness test value was achieved.
3. Result and discussion
3.1. Optical microstructure observation
The high-power microstructure of 10B21 boron steel from the sample center to the boundary before heat treatment is shown in Fig. 1(a). It can be seen from the Fig. 1(a) that the grains near the center are elongated and evenly distributed along the stretching direction, and the grains near the boundary which is shown in the true-line are distributed laterally, and are concentrated in the middle at the rightmost edge. This grain distribution is caused by the early spinning. In the process of wire rolling, the outer surface of the workpiece is squeezed, forming threads. The microplastic deformation occurs under the action of extrusion pressure, which changes the grain distribution and affects the mechanical properties. Fig. 1(b) is the enlarged image of the white box in Fig. 1(a). It shows the grains in this region are distributed in strips. Combined with the analysis of the iron-carbon phase diagram, the black block area indicated by the arrow in the figure is pearlite structure, and the grain indicated by the dotted arrow is ferritic structure. It is proved that the microstructure of the material before heat treatment is mainly ferritic and pearlite, and the microstructure of the two phases is evenly distributed.
Fig. 1(c) is the high power microstructure of 10B21 boron steel after heat treatment. As it is shown, the grain of the material changed greatly after heat treatment. The original ferrite and pearlite changed into strip martensite after quenching and tempering, and the lath structure was very fine. The plates are interleaved, and the microstructure has good strength and toughness, and the resistance to crack propagation is larger. The higher the delay sensitivity, the higher the fastener strength. At the same strength, low carbon martensitic steel has lower delayed fracture sensitivity than 40Cr medium carbon alloy steel. This is closely related to the special structure and substructure of low carbon martensite [15-16].
Fig. 1Optical micrographs of 10B21 steel
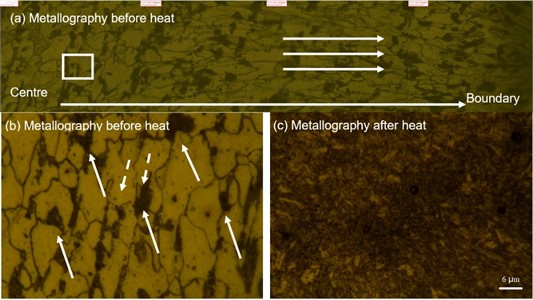
3.2. Mechanical properties of 10B21 steel
3.2.1. Hardness
Fig. 2 shows the microhardness of 10B21 boron steel before and after heat treatment under 0.3 kgf load. As shown in the graph, the hardness value of the material before heat treatment is about 206 HV, and the hardness value near the edge of the sample is slightly larger, which is related to the sample being rolled or rolled before heat treatment. Plastic deformation occurs in the part of the sample treated with wire rolling or wire rolling, resulting in changes in the surrounding tissues, as shown in Fig. 1(a), resulting in slightly higher hardness value. Its hardness after heat treatment is about 302HV. It can be seen that the hardness of 10B21 boron steel increases significantly after heat treatment. This is because the material structure changes from ferrite + pearlite to martensite after heat treatment [16].
Fig. 2Hardness of 10B21 boron steel before and after heat treatment
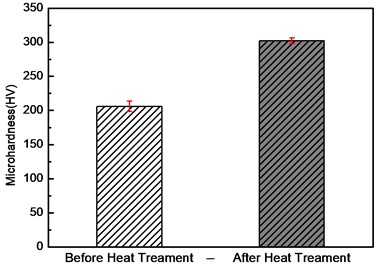
3.2.2. Tensile test
Fig. 3 shows the - (stress - strain) curves of 10B21 bolt fasteners in two different states after tensile test at room temperature, and Table 1 shows the relevant mechanical properties. It can be seen from the table that the tensile strength and yield strength of 10B21 samples after heat treatment are higher, and the plasticity increases with the increase of elongation. This is because the B element exists in the steel in the form of solid solution, which improves the hardenability of the alloy steel of this kind of steel and makes the carbon-boron steel obtain better comprehensive mechanical properties after tempering.
After being heated to 950 °C and water quenched, slatted martensite is obtained, and its substructure is high-density dislocation [17]. At 200 °C tempering, because of the carbon atoms image dislocation segregation and eliminating part of the internal stress, so the plasticity gradually increased. The slatted martensite got good toughness, mainly due to the presence of participating austenite between the slats, although its amount is small, its role cannot be ignored [18].
Fig. 3Tensile curves of two different states of 10B21 steel
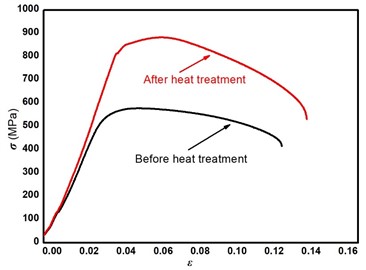
Table 1Tensile strength and elongation of 10B21 without and with heat treatment
10B21 Boron steel | Yield strength (MPa) | Strength of extension (MPa) | Elongation (%) |
Before heat treatment | 575 | 580 | 11.43 |
After heat treatment | 865 | 882 | 15.02 |
4. Conclusions
In this study, the optical microstructure and mechanical properties of 10B21 steel are analyzed by before and after heat treatment. Among them, WA-600 universal testing machine and HV-1000A microhardness tester are used to investigate the mechanical properties.
The result shows that the structure of 10B21 cold heading forming fasteners are changed after heat treatment (quenching and tempering). The microstructure changed from ferrite and pearlite to tempered martensite. Meanwhile, the mechanical and chemical properties of the workpiece are changed. The hardness and tensile strength of 10B21 cold heading forming fasteners were significantly improved after heat treatment.
References
-
E. Z. Cao, S. G. Song, Z. J. Chen, X. Q. Yang, W. Li, and J. Shi, “Production of 10B21 cold heading steel for high strength fasteners,” (in Chinese), Henan Metallurgy, Vol. 28, No. 4, pp. 32–34, 2020.
-
S. Gélinas and C. Blais, “Characterisation of the mechanisms taking place during liquid phase sintering of PM boron steels with the help of artificial intelligence,” Powder Metallurgy, Vol. 66, No. 1, pp. 29–42, Jan. 2023, https://doi.org/10.1080/00325899.2022.2055888
-
Y. B. Pei, T. J. Wang, Q. L. Ge, and X. H. Qu, “Study on microstructure and properties of high borated steel,” (in Chinese), Powder Metallurgy Industry, Vol. 32, No. 5, pp. 52–55, 2022, https://doi.org/10.13228/j.boyuan.issn1006-6543.20210038
-
V. Bhalerao, S. S. Lakade, and A. Borgaonkar, “The effect of boron nitride nanoparticles on 100Cr6 steel’s mechanical and tribological properties after vacuum heat treatment,” Materials Today: Proceedings, Vol. 77, pp. 941–945, 2023, https://doi.org/10.1016/j.matpr.2022.12.062
-
Murathan, K. Kocatepe, and M. Erdogan, “Effect of ultra-high boron additions on microstructure and mechanical properties on high chromium steel,” Materials Testing, Vol. 64, No. 12, pp. 1836–1842, Dec. 2022, https://doi.org/10.1515/mt-2022-0237
-
Z.-J. Wang, Y.-W. Li, S.-B. Gao, G.-D. Wang, and H.-T. Liu, “Enhanced strength-ductility synergy in brittle high borated steel by tailoring strain hardening behaviour,” Scripta Materialia, Vol. 230, p. 115398, Jun. 2023, https://doi.org/10.1016/j.scriptamat.2023.115398
-
L. D. Dong and W. Q. Chen, “Effect of cooling rate on microstructure transformation of 10B21 cold-heading steel containing boron,” (in Chinese), Heat Treatment of Metals, Vol. 35, No. 11, pp. 30–33, 2010, https://doi.org/10.13251/j.issn.0254-6051.2010.11.007
-
Q. Tang, P. C. Guo, H. Luo, H. H. Ma, L. Q. Zhang, and L. X. Li, “Hot deformation behavior and hot processing map of hot-formed 22mnb5 ultra-high strength steel for automobile body,” (in Chinese), Materials Reports, Vol. 18, pp. 1–14, 2023.
-
G. J. Yang and H. M. Zhao, “Research on hot stamping forming technology of ultra high strength boron steel sheet,” (in Chinese), Nonferrous Metals Engineering, Vol. 12, No. 9, pp. 177–178, 2022.
-
S. Lysykh, V. Kornopoltsev, U. Mishigdorzhiyn, Y. Kharaev, and Z. Xie, “Evaluation of wear resistance of aisi l6 and 5140 steels after surface hardening with boron and copper,” Lubricants, Vol. 11, No. 2, p. 48, Jan. 2023, https://doi.org/10.3390/lubricants11020048
-
J. Ren et al., “Effect of boron addition on the oxide scales formed on 254SMO super austenitic stainless steels in high-temperature air,” Metals, Vol. 13, No. 2, p. 258, Jan. 2023, https://doi.org/10.3390/met13020258
-
M. E. Palmieri and L. Tricarico, “Process window definition to predict mechanical properties of press hardened parts of Boron steel with tailored properties,” in Key Engineering Materials, Vol. 926, pp. 635–644, Jul. 2022, https://doi.org/10.4028/p-2c81z5
-
D. Liu, J. Yang, and Y. Zhang, “Effect of boron content on microstructure and impact toughness in CGHAZ of shipbuilding steel plates with Ca deoxidation,” Steel Research International, Vol. 94, No. 3, p. 22002, 2023.
-
S. L. Li and S. P. Ruan, “Effect of Si content on structure and properties of 10B21 steel hot-rolled coil,” (in Chinese), Special Steel, Vol. 41, No. 2, pp. 15–17, 2020.
-
Y. Hu, “Effect of boron on mechanical properties and fatigue life of spring steel for automobile suspension,” in Journal of Physics: Conference Series, Vol. 2437, No. 1, p. 012064, Jan. 2023, https://doi.org/10.1088/1742-6596/2437/1/012064
-
X. M. Zhang, “Heat treatment process for fastener in carbon boron steel,” (in Chinese), MW Metal Forming, Vol. 21, pp. 33–35, 2009.
-
M. S. Mohamed, W. Khalifa, and M. G. Mahmoud, “Failure analysis of alloy boron steel bolts in steel structure assembly,” Journal of Failure Analysis and Prevention, Vol. 23, No. 1, pp. 88–98, Feb. 2023, https://doi.org/10.1007/s11668-022-01552-0
-
M. E. Palmieri and L. Tricarico, “Comparison of flow behaviors at high temperature of two press hardening boron steels with different hardenability,” Metals, Vol. 12, No. 11, p. 1935, Nov. 2022, https://doi.org/10.3390/met12111935
About this article
The authors would like to acknowledge the Science and Technology Research Program of Chongqing Municipal Education Commission (No. KJQN202203003).
The datasets generated during and/or analyzed during the current study are available from the corresponding author on reasonable request.
The authors declare that they have no conflict of interest.